Several years ago, stage rigger Tom Heemskerk posed a question on Spartalk: How does a block-and-tackle provide mechanical advantage? That is, what mechanism is at work, such that you can pull on a rope with a certain amount of force at one end, and move a corresponding or greater amount of weight at the other? It was a question that many of us -- including yours truly -- thought we knew the answer to. After all, the pulley is a basic piece of machinery, something we learned about as children.
But when we set about answering Tom's question, our theories wound up being wrong, or meaningless, or both. And we were in good company, as no shortage of textbooks, handbooks, and dictionaries would describe what happens ("Mechanical advantage is equal to the number of parts of rope coming from the moving block"), but would never say why. Everyone could say that there was power in a block-and-tackle, and most of us could calculate how much power was gained in a given configuration, but no one could say where it was coming from. It was a weird moment, as we confidently set about describing this simple machine, only to realize that we actually hadn't a clue.
Tom finally took pity on us, and gave the answer, which he had, in turn, gotten from a naval architect in a pub somewhere. He (Tom) actually came to Port Townsend during a Wooden Boat Festival to demonstrate the solution, and all who were there had a wonderful feeling of profound gestalt as we watched the explanation unfold.
I resolved then and there to publish a written version here, in Fair Leads, but because doing so involved sitting down and making some extremely simple drawings, I've been putting it off all this time. Sorry. So here at last is the insight.
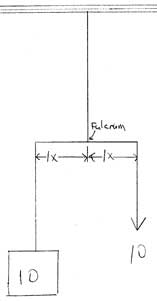 |
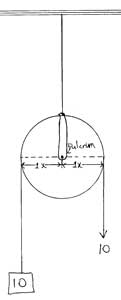 |
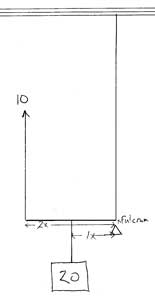 |
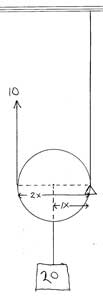 |
Fig. 1 |
Fig. 2 |
Fig. 3 |
Fig. 4 |
A block and tackle is either a first class or second class form of lever, depending on whether the block is moving or fixed in place. If the block is fixed, it is a lever of the first class, in which the fulcrum lies between the load and the force. If you look at fig.1, a bar, suspended by its middle, has a ten pound force pulling down on one end, and a ten pound load pulling down on the other. The fulcrum is between force and load, so this is a first class lever. Think of it as a seesaw lever. And since the fulcrum is right in the middle, a load and force of equal amounts are balanced on the fulcrum; the mechanical advantage is 1:1.
Now look at fig.2, which shows a block suspended by its axle. The axle is the fulcrum, and the dotted lines show where the bar was in fig.1. Because a pulley's axle is always centered in the sheave a block that is fixed in place always requires a force equal to the load, just to hold things steady, and a force greater than the load to move the load; the pulley just redirects the force, and the advantage is always 1:1.
Ah, but look at fig.3, in which the load is between the force and the fulcrum. This is a second class lever, a "can opener" lever, and in this case the force is twice as far from the fulcrum as the load is. This means that half of the load rests on the fulcrum, so we only have to pick up half the load with the force. Voila, a 2:1 mechanical advantage.
Finally, in fig.4, we have a load suspended from a block. The block, in turn, is suspended from a point overhead -- not by its axle, but by a line passing around the sheave. Yup, the same 2:1 mechanical advantage.
That is why we count the parts coming out of the moving block(s), because for each part we gain one part of purchase. That is also why we don't count any parts coming out of a fixed block, because all of those parts simply represent redirected leads.
There's a bit more to mechanical advantage gained with block-and-tackle, like the effect of angles, of friction, of compounded purchases, etc. But the basic mechanism is always the same elegant application of leverage.